Cancer has been described as the most common genetic disease. This doesn’t necessarily mean it’s hereditary – usually the genetic mistakes that cause cancer arise in the body’s organs or tissues and can’t be inherited. We’re continually learning of new cancer-causing genetic mistakes.
If we think of genes as words spelling out the instructions for our bodies to function, there are different types of mistakes or “typos” that can cause cancer. Some of these are like spelling mistakes – an incorrect letter or two. The mistakes I’ll be talking about here involve whole words (or lots of them). For example one or more copies of a word are added – ” very big” becomes “very very big” – extra copies of a cancer gene (we call them oncogenes) can cause or accelerate cancer growth. Or if a word is lost – “don’t grow” becomes “grow” – this illustrates loss of a tumour suppressor gene.
I described the breakage-fusion-bridge cycle a few weeks back. The BFB cycle was a theory developed from studies with maize, but it also applies to some cancers. This is an example of basic research, inspired by curiosity but eventually being useful in ways we never imagined. If you look back at that post it shows how the BFB cycle can cause gain or loss of genes. If a cancer gene is multiplied, or if a tumour suppressor gene is in the part that’s lost, the cell can gain a growth advantage over other cells, which is part of the process causing cancer.
Here’s an example.
Cancer cell lines are cancer cells that can be grown indefinitely in the laboratory. I’ve just published a paper on HEL, which is a leukaemia cell line. It’s popular for studying how cells make globin (molecules in red blood cells that help us process the air we breathe).
The chromosomes of HEL are very abnormal. I’ve used a combination of techniques to show how the chromosomes are reorganised as well as which parts have been lost, gained and amplified. It’s very complicated.
One of the gene abnormalities in HEL is amplification of the JAK2 gene. JAK2 is a well-known cancer gene that is often abnormal in blood cancers. The normal gene can be mutated to become a cancer gene, for example by a “spelling mistake” in the DNA. By adding extra copies of this abnormal gene the effects can be magnified. This is known as gene amplification. There are a few cancer genes that are commonly amplified in cancers.
To cut a long story short, JAK2 is amplified in the HEL cell line. And a nearby tumour suppressor gene (CDKN2A) has been lost. But only by looking at the chromosomes does the reason become clear. Some detective work tells us that there were some breakage-fusion-bridge events. I won’t go into the detail – if you’re interested it’s in the paper. But we have chromosomes whose ancestors had two centromeres, and if we use a DNA tag for the region between the centromeres we can see “stripes”.
Here’s an example from HEL that shows DNA amplified by the BFB cycle – we can show where a gene is on the chromosomes by labelling it with a fluorescence tagged DNA “probe”. The striped pattern reminds us of the yellow dots in the modelling clay demonstration:

The red is DNA that’s normally at one end of some of the chromosomes. The stripes tell us that the end of a chromosome (22) is in the middle of these chromosomes and there are extra copies. It’s a strong clue that BFB cycles were involved and the ancestral chromosome had two centromeres.
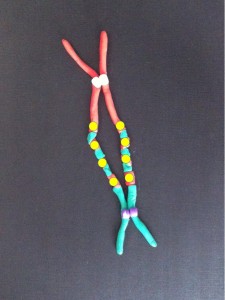
The new chromosome with four copies of the yellow gene courtesy of the breakage-fusion-bridge cycle.
Recently JAK2 amplification was also reported in triple-negative breast cancer. Triple negative means that three well-known genetic causes of breast cancer are not present. So finding JAK2 amplification would help explain the cause of some triple-negative breast cancers, and could help work out an effective treatment. Perhaps this JAK2 amplification is sometimes caused by BFB cycles. Without looking at the layout of the abnormal chromosomes we may never know.
To end this story, here’s Bruce Mercer’s cartoon diagram showing the BFB cycle in HEL: http://emph.oxfordjournals.org/content/2013/1/225/F6.expansion.html
Related articles
- A Constellation in the Chaos of Cancer Chromosomes (thealmagest.com)